User:SPat/Experimental condensed matter physics
Experimental condensed matter physics is the branch of physics that involves using experimental probes and methods to address problems in condensed matter physics. Experimental condensed matter physics is an active area of research in physics laboratories worldwide. Experimental condensed matter physics is one of the core research areas under the Basic Energy Sciences scheme of the U. S. Department of Energy.
History[edit]
Materials synthesis[edit]
Crystal growth[edit]
Nanofabrication[edit]
Thin film deposition[edit]
Molecular beam epitaxy[edit]
Analytical techniques[edit]
Cryogenic and high magnetic field environments[edit]
Quantum oscillations[edit]

Quantum oscillations refers to a variety of techniques that are based on the phenomenon of oscillations of various physical parameters with increasing magnetic field. The presence of magnetic fields causes the electronic states in materials to split into Landau levels. Increasing magnetic fields thus cause observables such as the electronic density of states, magnetic dipole moment (the de Haas-van Alphen effect), and resistivity (the Shubnikov-de Haas effect) to oscillate, generating a map of the Fermi surface of materials.
Quantum oscillations in high-temperature cuprate superconductors such as YBCO have provided important evidence for non-Fermi liquid behavior in strongly correlated electron systems.[1] The National High Magnetic Field Laboratory in Tallahassee, Florida, the Pulsed Field Facility in Los Alamos, New Mexico, both in the United States, and the National Laboratory for Intense Magnetic Fields in Toulouse, France are some of the laboratories worldwide involved in quantum oscillations experiments.
Nernst effect[edit]
Susceptibility[edit]
SQUID magnetometry[edit]
Transport properties[edit]
Electrical transport[edit]
Hall effect[edit]
Hall effect refers to the phenomenon where if a current carrying conductor is placed in a perpendicular magnetic field, a potential difference is formed in the conductor on edges that are perpendicular to both the current and the magnetic field. The resultant potential difference is called the Hall voltage, and it's ratio to the transverse current is called the Hall coefficient. The effect is named after Edwin Hall, who discovered it in 1879. The quantum Hall effect refers to the Hall effect that occurs in two-dimensional electron gas systems where the magnetic fields are high enough, or temperature is low enough for Landau levels to be prominent.
Measurements of the Hall effect give information about topological properties of Fermi surfaces in materials. For example, the integer quantum Hall effect is a direct consequence of Landau quantization. The fractional quantum Hall effect, discovered in 1982 is a consequence of strong Coulomb interactions between electrons altering particle dynamics. Measurement of half-integer Hall effect has been reported in single-layer graphene, indicative of properties such as electron-hole degeneracy and vanishing carrier mass.[2][3]
Thermal transport[edit]
Scattering techniques[edit]
X-ray scattering[edit]
Neutron scattering[edit]
Microscopy and optical techniques[edit]
Scanning electron microscopy[edit]
Fourier transformed Infrared spectroscopy[edit]
Angle-resolved photoemission spectroscopy[edit]
Angle-resolved photoemission spectroscopy (ARPES) is an experimental technique to measure electronic structure on the surface of solids. It is based on the phenomenon of photoemission, wherein photons from a light source excite electrons in materials to unbound states causing them to be emitted from the surface of the material. In ARPES, both the momentum and kinetic energy of emitted electrons can be measured. As the initial photon energy is known, and the photon momentum is usually negligible, this allows one to get information about the quantum state the electron was emitted from. Specifically, the intensity of the photocurrent is analyzed to generate the spectral function , which is related to the single particle Green's function .
ARPES has been one of the leading tools for studying quantum materials over the past decade. ARPES has been used to study the symmetry of the superconducting order parameter, and electronic phase competition in cuprate and Iron pnictide superconductors. ARPES spectra have produced direct evidence for the existence of Dirac cones in the band structure of graphene. Because ARPES provides surface measurments, it is ideally suited to verify some of the experimental predictions about topological insulators which have distinct surface charge carrier states.[4]
X-ray spectroscopy[edit]
Ellipsometry[edit]
Raman spectroscopy[edit]
Local probes[edit]
Atomic force microscopy[edit]
Scanning tunneling microscopy[edit]
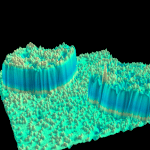
Scanning tunneling microscopy (STM) is an imaging technique based on the concept of tunneling in quantum mechanics. The technique works by bringing a conducting "tip" very close to the surface of the material being observed, in a controlled fashion. The tip and the material are maintained at a constant potential difference. The resulting current from electrons tunneling across the narrow gap is measured in order to map out the local electronic density of states. Scanning tunneling microscopes can routinely reach resolutions of less than 0.1nm, and hence, are often able to image individual atoms on a surface. STM only works with materials that are conducting.
In addition to many applications in biology and nanoscale engineering, scanning tunneling microscopes are an important tool for studying material properties from the point of view of condensed matter physics.[5] STM measurements have been used to study the onset of superconductivity and the pseudogap phase in high temperature superconductors.[6] Spin polarized scanning tunneling microscopy, where the spin of STM electrons is measured, along with their charge, has been used to study magnetic domains in magnetically ordered systems.[7]
References[edit]
- ^ Nandkishore, Rahul; Metlitski, Max A.; Senthil, T. (1 July 2012). "Orthogonal metals: The simplest non-Fermi liquids". Physical Review B. 86 (4): 045128. doi:10.1103/PhysRevB.86.045128.
- ^ Macdonald, A. H. (1994). "Introduction to the Physics of the Quantum Hall Regime". arXiv:condmat/9410047.
- ^ Zhang, Yuanbo; Tan, Yan-Wen; Stormer, Horst L.; Kim, Philip (10 November 2005). "Experimental observation of the quantum Hall effect and Berry's phase in graphene". Nature. 438 (7065): 201–204. doi:10.1038/nature04235. PMID 16281031. Retrieved 5 August 2013.
- ^ Lu, Donghui; Vishik, Inna M.; Yi, Ming; Chen, Yulin; Moore, Rob G.; Shen, Zhi-Xun (1 March 2012). "Angle-Resolved Photoemission Studies of Quantum Materials". Annual Review of Condensed Matter Physics. 3 (1): 129–167. doi:10.1146/annurev-conmatphys-020911-125027.
- ^ Coleman, Piers (15 June 2011). "Condensed-matter physics: Microscopy of the macroscopic". Nature. 474 (7351): 290–291. doi:10.1038/474290a. PMID 21677741.
- ^ Parker, Colin Vanderslice (2011). Exploring Superconductivity and Pseudogap Using High Resolution Scanning Tunneling Microscopy (PDF). Princeton University.
- ^ Wulfhekel, Wulf; Kirschner, Jürgen (1 January 1999). "Spin-polarized scanning tunneling microscopy on ferromagnets" (PDF). Applied Physics Letters. 75 (13): 1944–1946. doi:10.1063/1.124879. Retrieved 4 August 2013.